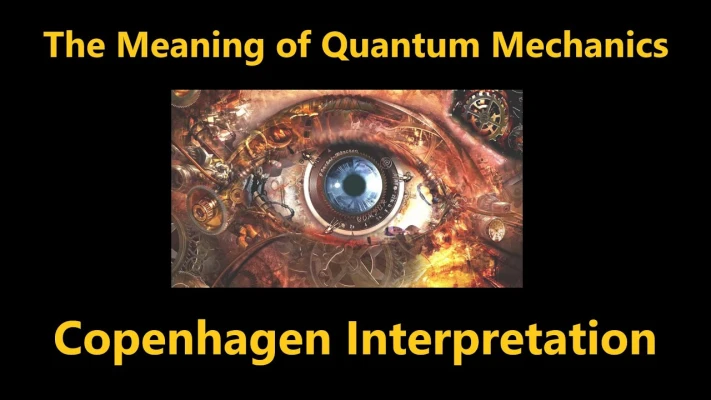
The Copenhagen interpretation is one of the most well-known and widely discussed explanations of the nature of quantum mechanics, developed in the early 20th century. It was mainly formulated by physicists Niels Bohr and Werner Heisenberg in the 1920s, both of whom were based in Copenhagen, Denmark, at the time. This interpretation forms the foundation of modern quantum theory, providing a framework for understanding how quantum systems behave.
In the Copenhagen interpretation, quantum mechanics is seen as a mathematical tool for predicting the outcomes of experiments rather than a direct description of reality. The interpretation is both influential and controversial, leading to many debates about the nature of reality and measurement in the quantum world.
Key Concepts of the Copenhagen Interpretation
1. Wave-Particle Duality
One of the central ideas of quantum mechanics is wave-particle duality, the notion that particles like electrons, photons, and other subatomic particles exhibit both particle-like and wave-like behaviors. In the Copenhagen interpretation, this duality is a fundamental feature of nature.
For instance, electrons can act as waves when they are not observed, spreading out across space. However, when measured or observed, these same particles behave like particles, having a definite location. This is most famously demonstrated in the double-slit experiment, where the behavior of particles changes depending on whether or not they are being observed.
2. The Role of the Observer
One of the most striking aspects of the Copenhagen interpretation is the role that measurement or observation plays in determining the state of a quantum system. Prior to measurement, quantum objects are described by a wave function, which provides a probability distribution for all possible outcomes of an observation. This wave function represents a “superposition” of all possible states the system could be in.
However, once a measurement is made, the wave function “collapses” into a single, definite state. This means that the act of observing forces the system to take on a specific outcome. Before measurement, the system exists in a superposition of states, but after measurement, it is confined to a single outcome.
This idea is encapsulated in Heisenberg’s Uncertainty Principle, which states that certain pairs of properties, like position and momentum, cannot be known simultaneously with perfect precision. The act of measuring one property disturbs the other, highlighting the interaction between observation and the system.
3. Probability and Indeterminacy
The Copenhagen interpretation introduces a fundamental element of indeterminacy into the nature of quantum mechanics. Unlike classical physics, where systems evolve in a deterministic way (where the future state of a system can be predicted if its current state is known), quantum mechanics is inherently probabilistic.
When dealing with quantum systems, we cannot predict with certainty the outcome of a particular event, only the probabilities of different outcomes. The wave function describes these probabilities, but the actual result of a measurement is random and unpredictable. This introduces the concept of quantum indeterminacy, where the future state of a system is not predetermined but based on probability.
4. Complementarity Principle
The complementarity principle, introduced by Niels Bohr, is a key idea in the Copenhagen interpretation. It asserts that different aspects of a quantum system (such as wave and particle behaviors) are complementary to one another. They are mutually exclusive but both are necessary to fully understand the system. For example, light behaves as a wave in some experiments and as a particle in others. These two descriptions are complementary because they provide a complete picture of light’s behavior.
In the Copenhagen interpretation, this complementarity extends beyond the wave-particle duality to all aspects of quantum systems. The behavior of quantum particles depends on how they are measured, and no single measurement can capture all the properties of a quantum system.
Schrödinger’s Cat: The Paradox of Superposition
One of the most famous thought experiments related to the Copenhagen interpretation is Schrödinger’s Cat, proposed by physicist Erwin Schrödinger in 1935. This paradox was designed to illustrate the apparent absurdity of the Copenhagen interpretation’s reliance on observation and wave function collapse.
In the thought experiment, a cat is placed in a sealed box with a vial of poison that is set to be released if a certain quantum event (such as the decay of a radioactive atom) occurs. According to the Copenhagen interpretation, before the box is opened and the system is observed, the cat exists in a superposition of both alive and dead states. It is only when the box is opened, and the cat is observed, that the superposition collapses, and the cat is either found to be alive or dead.
Schrödinger’s paradox highlights the challenge of applying quantum mechanics to macroscopic objects and led to many philosophical discussions about the nature of measurement and reality.
Controversies and Alternatives
While the Copenhagen interpretation remains one of the most widely accepted interpretations of quantum mechanics, it has not been without its critics. Some argue that the idea of wave function collapse is an incomplete explanation, while others take issue with the role of the observer in determining reality.
1. Einstein’s Objection
Albert Einstein was one of the most vocal critics of the Copenhagen interpretation. He famously said, “God does not play dice,” reflecting his discomfort with the inherent randomness and indeterminacy of quantum mechanics. Einstein believed that there must be underlying hidden variables that determined the outcomes of quantum events, even though these variables had not yet been discovered.
In response, Bohmian Mechanics (also called the pilot-wave theory) was developed as an alternative interpretation. It suggests that particles have definite positions and velocities at all times, but these are guided by a “pilot wave.” This interpretation eliminates the need for wave function collapse and restores determinism to quantum mechanics.
2. The Many-Worlds Interpretation
Another alternative to the Copenhagen interpretation is the Many-Worlds Interpretation, developed by Hugh Everett in 1957. In this theory, all possible outcomes of a quantum event occur, but each outcome takes place in a separate, parallel universe. In contrast to the Copenhagen interpretation, where the wave function collapses into one reality, the Many-Worlds interpretation suggests that the wave function never collapses. Instead, the universe splits into different branches, each representing a different outcome.
The Copenhagen Interpretation’s Legacy
Despite its controversies, the Copenhagen interpretation has played a crucial role in shaping our understanding of quantum mechanics. It remains the most widely taught interpretation in textbooks and has been the foundation of countless experiments and technological advancements.
Quantum technologies like quantum computing, quantum cryptography, and quantum teleportation are based on principles consistent with the Copenhagen interpretation, such as superposition and entanglement. As quantum research continues to evolve, the Copenhagen interpretation remains a critical framework, though alternative interpretations continue to offer new perspectives.
Conclusion
The Copenhagen interpretation is not just an explanation of quantum mechanics but also a philosophical lens through which we view the nature of reality. By suggesting that the universe operates probabilistically and that observation plays a key role in determining outcomes, it challenges our classical understanding of the world.
Even though the interpretation has faced criticism and spurred the development of alternative theories, its influence on both science and philosophy remains profound. Whether or not future discoveries will validate or refute the Copenhagen interpretation, it will continue to be a central chapter in the story of quantum mechanics.